Superhydrophobic Tracks: Guided Transport of Droplets
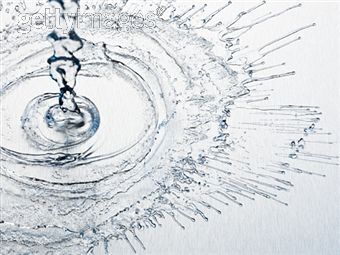
Recent work by research groups including Mertaniemi et al.has shown that superhydrophobic tracks can be used for fast and simple transport of water droplets in microfluidic devices.
The wetting properties of the superhydrophobic surfaces were characterised by measuring dynamic contact angles using an optical tensiometer. These are scientific instruments are used to measure surface tension, interface tension and contact angles.
This article describes how the dynamic contact angle was measured by changing
the droplet volume on superhydrophobic surfaces.
A surface is defined as ‘superhydrophobic’ if the contact angle of water is larger than 150° and water droplets readily slide off the surface if tilted slightly.
Two factors are required to create a superhydrophobic surface. Firstly, the surface must have suitable roughness at micro and nanoscales. Secondly, the surface must have a hydrophobic surface chemistry
Only when the contact angle hysteresis (difference between the advancing and receding contact angle) is negligible can the wetting properties of a surface be characterised by a static contact angle, which is measured by placing a droplet on the surface and optically determining the contact angle.
For rough surfaces, dynamic (advancing and receding) contact angles need to be measured, since a static contact angle can take any value between the advancing and receding ones.
In the study, the superhydrophobic tracks were prepared in metal plates by milling or laser cutting and in silicon by ion etching. The metal surfaces were coated using a combination of silver microstructure and a fluorinated thiol surfactant, and the silicon wafers were coated with a fluoropolymer in CF3 plasma.
Wetting properties of a superhydrophobic copper surface were characterised using a Theta optical tensiometer from Attension (part of the Biolin Scientific Group, a global provider of analytical instruments).
First, a 1-μl droplet was applied on the surface, and the needle was lowered into the drop so that the tip was about at the midway of the droplet height.
The volume of the droplet was slowly increased to 2 μl, at a drop rate of 0.05 μl/s. In order to minimise hysteretic effects, the addition of water was stopped for 30 seconds before starting the contact angle measurement.
To measure the advancing contact angle, the volume of the droplet was increased from 2μl to 10 μl at 0.05 μl/s, recording images at 0.6 frames per second.
Next, the droplet volume was increased to 15 μl and decreased back to 11 μl. After this, the droplet volume was decreased slowly to 10 μl.
In order to minimise hysteretic effects, the removal of water was stopped for 30 seconds before starting the receding contact angle measurement.
The receding contact angle was measured by decreasing the volume at a rate of 0.05 μl/s, starting at a drop volume of 10 μl. Images were recorded at 0.6 frames per second until the drop lost contact with the surface.
A similarity of contact angle values of the zinc and copper surfaces was expected, since both surfaces were coated using the same method. However, the larger variance of receding contact angles observed for the zinc surface suggests that the coating had some defects.
The very small contact angle hysteresis of the silicon surface makes it the optimal choice for applications where extremely high mobility of water droplets on a surface is desired.